C-Factor of Thermal Conductance
C-factor is one of the overall thermo-physical performance metrics for opaque construction assemblies of a building envelope. It is defined as the time rate of steady-state heat flow through the unit area of a material or construction induced by a unit (1 Kelvin) temperature difference between the body surface. C-factor is identified as a measure of thermal conductance instead of resistance therefore it has the unit of measurement as Btu/h. ft^2.F in IP system and W/m^2K in SI system. Right from the outset, C-factor seems to be very similar to the U-factor (overall coefficient of thermal transmission) which has the same unit of measurement (W/m^2K). While many design professionals are well accustomed to evaluating and using U-factor to describe the thermal performance of their envelope designs, the C-factor is not a well-known metric which can create confusion about its application and interpretation as well. The question is what is up with the C-Factor?
C-factor is like U-factor in describing the heat flow characteristics, but C-factor does not include the resistances of the interior air film, exterior air film or the effect of the earth. All these factors which are accounted for the U-factor calculations are totally removed for simplification purposes. C-factor does not include air or soil films and as opposed to U-factor; it is only applicable to below-grade wall assemblies but not to any other assembly types. Below-grade walls are the basement walls and defined as the portion of wall in the building envelope that is entirely below the finish grade and in contact with the ground. So, when it comes to quantitatively evaluating the thermal resistance properties of basement walls, the inside-outside air film resistances are ignored (there is no air film next to the exterior of surface of a basement wall anyway).
For instance, considering a below-grade wall assembly with the following material layers from outside to inside:
(1) Continuous Insulation (Ci) (R1 = 1.76 m^2K/W)
(2) 12” Solid grouted CMU (R2 = 0.30 m^2K/W)
(3) 1.5” Wood furring 24” o.c. with air gap without insulation (R3 = 0.22 m^2K/W)
Note: Continuous insulation (Ci) is required for below-grade walls of buildings located at Climate Zone 4-to-8 according to the ASHRAE 90.1 energy standard.
This wall assembly will have C-factor of (1/R1+R2+R3 = 1/1.76+0.30+0.22 = 1/2.29) 0.44 W/m^2K. As you see there is no air or soil film resistances factored into the calculation here. Therefore, for the same type of wall assembly the U-factors will always be smaller than the C-factor. Larger thermal conductance indicated by the C-factor can be viewed as an adverse effect, but we need to thing that below-grade walls are always protected by the convective heat loss impacts (of hot or cold air movements) as they are in contact with the earth.
When it comes to defining below-grade walls with the C-factor method in building performance modeling, there exists yet another fundamental difference between the well-known U-factor and the C-factor. An energy modeler can create an above-grade (external) wall assembly by building up an entire construction layer-by-layer using relevant materials from a program’s library. And the program can calculate the resultant U-factor for the given assembly make-up. That is usual method of performance evaluation as we know it. But when it comes to the C-factor method things are reversed! C-factor is taken as an input to the program that defines a specific below-grade wall assembly type. It is not an output metric of an arbitrarily defined assembly. Then how can we proceed with this method? Here is a step-by-step guide:
Step 1: Decide on the type of below-grade wall to be specified for the building design. You can discuss with the project architect, the design team for a quick feedback. A decision has to me made on the existence of framing or not, the existence of continuous insulation or not, the type of framing, the R-value of insulation alone.
Step 2: Find out the corresponding C-Factor for the below-grade wall under consideration from the ASHRAE 90.1 standard (e.g., ASHRAE 90.1-2016 Table A4.2.1).
For instance, according to Table A4.2.1, a below-grade wall without framing but with a continuous exterior thermal insulation with a rated R-value of 30 (R-30 h. ft^2.F/Btu) will have a C-factor of 0.032 Btu/h. ft^2.F.
Step 3: If needed convert this C-factor is IP units to SI units by simply multiplying it with 5.6782. In our example, the C-factor in SI units would be 0.181 W/m^2K.
Step 4: Enter the resultant C-factor to your energy simulation program to define the specific thermal characteristics of the below-grade wall that has been chosen for your building design.
There is no general rule for Step 4 as each simulation program can offer different settings for this type of data entry. Some programs offer alternative data entry for the C-factors instead of layer-by-layer definition of a wall assembly.
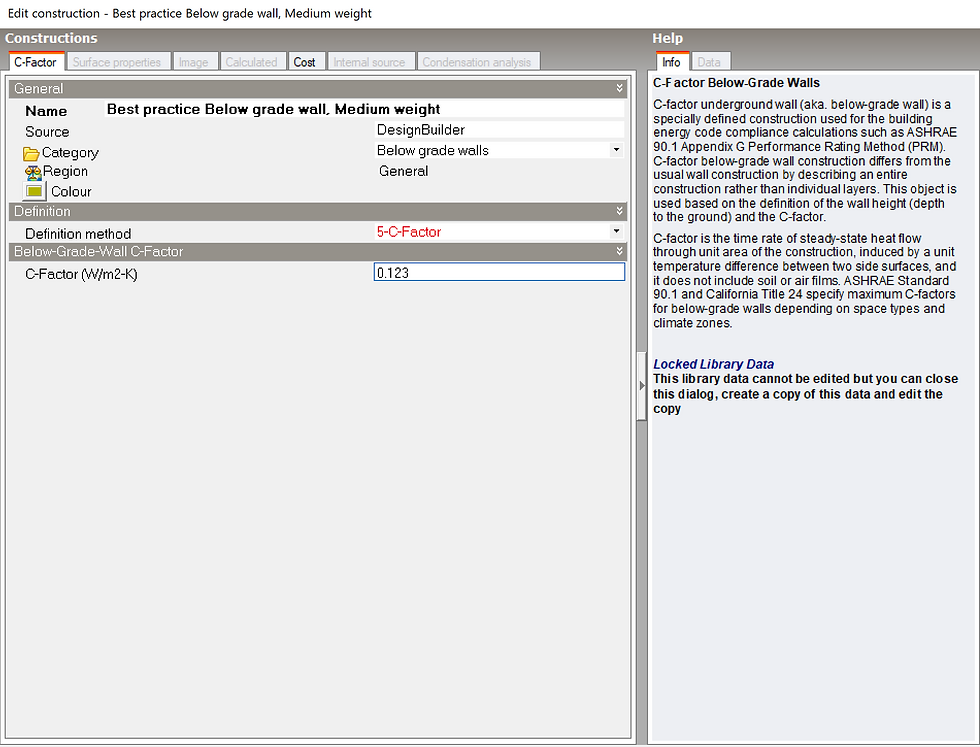
Design Builder v.6 interface with direct C-factor definition method.
Design Builder (DB) v.6 program which is a comprehensive interface to the U.S. DOE’s EnergyPlus simulation engine offers a direct entry of C-factor to characterize a below-grade wall assembly. Behind the interface of DB, EnergyPlus program considers the height (meter/ft) of the below-ground wall (depth from the surface) along with user defined C-factor and creates an equivalent -pseudo- construction assembly to model the ground heat transfer through the below-grade walls. C-factor-based constructions of the EnergyPlus are represented with two imaginary layers (a concrete wall with thermal mass and an insulation layer without a thermal mass). This simplified/approximated approach (instead of layer-by-layer definitions) saves user time to create special below-grade wall assembly using specialized EnergyPlus programs (Basement and Slab Tools) to generate monthly ground temperatures and calculate detailed heat flow characteristics through the assemblies.

It should be note that following the alternative method of C-factor input requires to update some other settings in the same model related to the Ground Temperatures. In DB case, Ground Temperatures can be updated within the location settings. The energy modeler needs to specific the source of the FC Factor method ground temperatures source which can be (1) Model Data which requires the modeler to enter the monthly temperatures manually or (2) Hourly weather file where DB extracts necessary data from the hourly weather data linked to the simulation model (0.5m depth set of monthly ground temperatures from the weather data file).
To be more accurate, (1) Model data source method can be followed. In such a case, the energy modeler needs to 12 data inputs for each month of the year. A quick solution to find this ground temperatures is to enter the monthly average daily outside temperatures delayed by three months. How to find the monthly average outside temperatures? The energy modeler can review the *.STAT file that comes along with the EPW weather data package and after scrolling down to the bottom part of the text-based STAT file, the Monthly statistics for dry-bulb temperatures table will be seen.

Based on this table, data can be entered into the DB interface by entering October temperatures in the place of January and continue till the end of 12 months cycle as seen below:
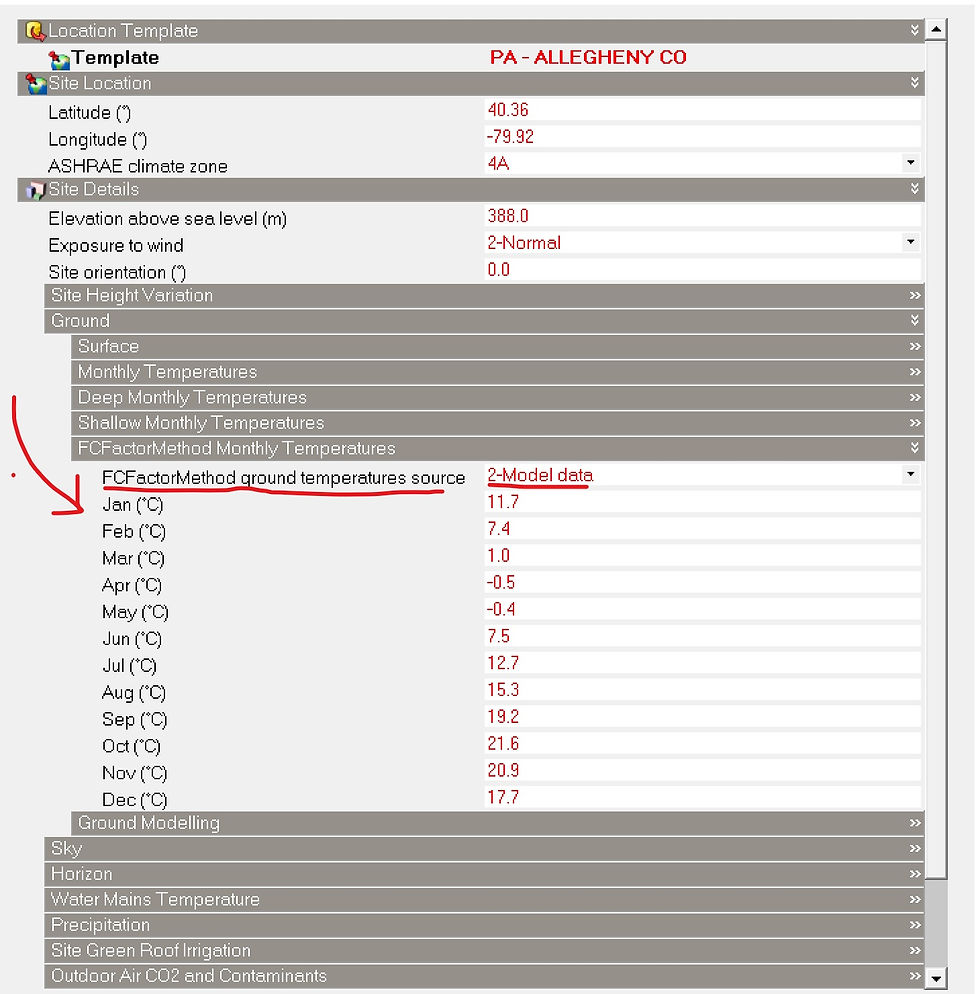
Another approach to apply the C-factors to a selected below-grade wall assemblies is to use the template libraries which are compatible with the ASHRAE 90.1 energy standard and which already contains entire assemblies classified based on their respective C-factors. For instance, after importing ASHRAE 90.1 compliant prototype construction library to the project, an IESVE-based model offers alternatives of below-grade wall types with specific C-factors that can selected by the energy modeler.
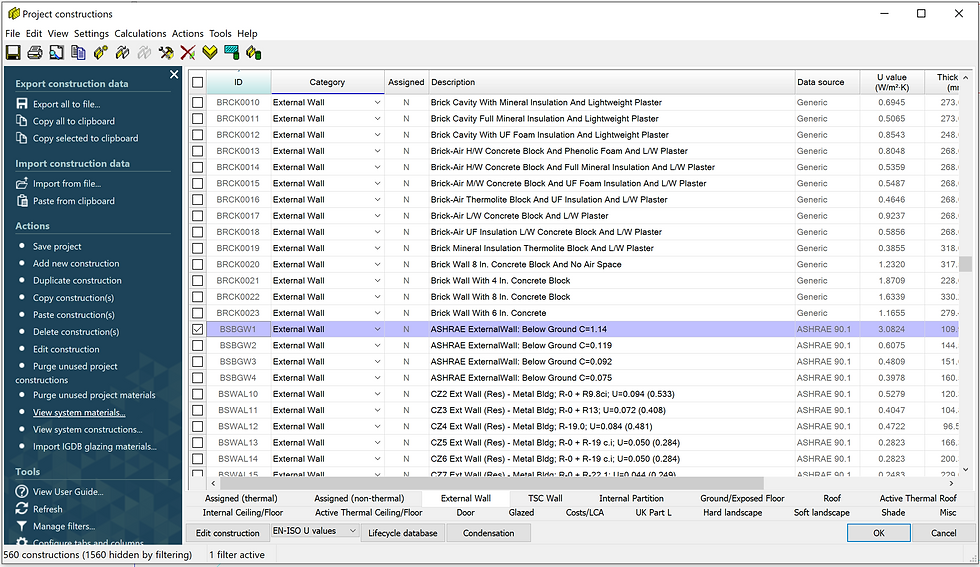
Energy modelers can also construct below-grade wall assemblies following the layer-by-layer method and then they can calculate the C-factors using the method that I explained above and check them against the required maximum C-factors levels of the ASHRAE 90.1 according to the climate zone of their building location. However, they need to find some detailed information about their simulation program’s methods of calculating the complex heat gain and loss characteristics through the under-ground/basement/below-grade walls that are in context with the earth surfaces and that are not exposed to solar radiation impacts and wind-induced convective forces. All of the methods explained here are approximated or pseudo methods for more detailed calculations, 2D transient thermal simulation models using finite-element approach (such as LBNL’s THERM program - https://windows.lbl.gov/software/therm).
Omer T. Karaguzel, PhD
Comments